Manuscript-Cerebral Cortex
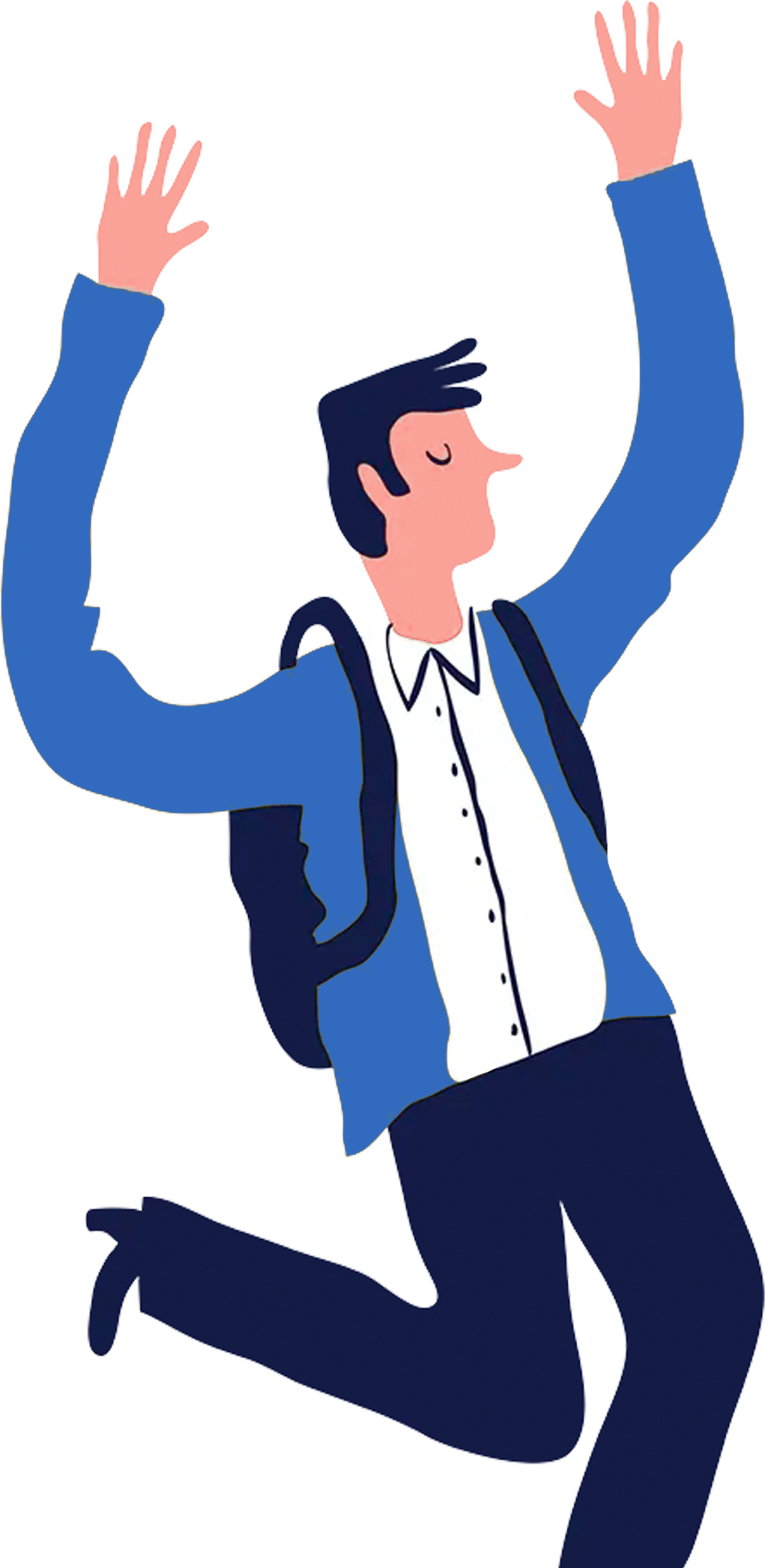
编者按
深圳大学外国语学院立足改革开放特区窗口、新时代先行示范区和国际化标杆城,永葆“闯”的精神,“创”的劲头,“干”的作风是我们的底色。学院秉承“语通中外,读懂世界”的院训,践行“铸中国发展之魂,育涉外核心之才”教育使命,立足湾区,胸怀祖国,放眼世界,借新时代外语学科创新转型之势,以新文科建设为指引,夯外国语言文学之基石,扩交叉学科之视野,拓外语赋能之潜力,以“外语+区域国别”“外语+国际传播”“外语+国际组织”“外语+国际经贸”“外语+……”等为抓手,坚持“厚基础、宽口径、差异化”发展路径,培养以“外语赋能”为特色的国际复合型人才。
本着“教研并重、科研强院”的理念,着力打造学科品牌,促进内涵式发展,我们特此推出“科研荟萃”专栏,宣介学院资深教授和青年才俊的代表性科研成果和重要科研学术活动,以期打造一个既“语通中外、读懂世界”又“启迪智慧、温润心灵”的学术空间站,从而促进科研学术的引领指导和交流融通,树立深大外院坚实的科研品牌。
作者介绍
陆烁,深圳大学特聘教授,语言学博士,英语系主任,博士生导师。研究方向:语言学及应用语言学、神经语言学、语言障碍的精准评估和矫正。
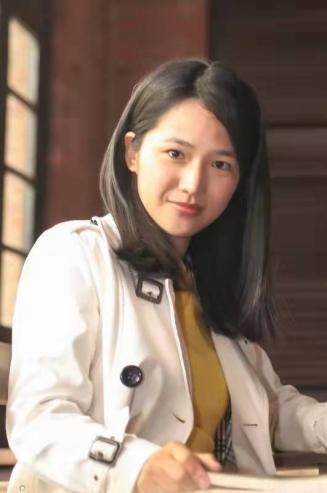
陆烁 教授
Main Manuscript for
Inside and beyond the visual cortex: Brain tumors induce highly sensitive plasticity in whole-brain neural functional networks
Within and beyond the visual cortex:
Brain tumors induce highly sensitive plasticity of visual processing in whole-brain neural functional networks
Jingwen Yang1,2†, Nijiati Kudulaiti 3,4,5,6†, Zelin Chen7, Leyan Gao1, N. U. Farrukh Hameed3,4,5,6, Rui Feng3,4,5,6†, Shuo Lu1,2†
†These authors contributed equally to this work.
1Teaching Laboratory of Neurolinguistics, Department of Chinese Language and Literature, Sun Yat-sen university, No. 135 Xingang West Road, Guangzhou, 510275, P. R. China
2Department of Clinical Neurolinguistic Research, Mental and Neurological Diseases Research Center, The Third Affiliated Hospital, Sun Yat-sen University, 600 Tianhe Road, Guangzhou, 510630, P. R. China
3Department of neurosurgery, Huashan hospital, Fudan university, No.12 Mid Wulumuqi Road, Shanghai, 200040, P. R. China
4Neurosurgical Institute of Fudan University, No.12 Mid Wulumuqi Road, Shanghai, 200040, P. R. China
5Shanghai Clinical Medical Center of Neurosurgery, No.12 Mid Wulumuqi Road, Shanghai, 200040, P. R. China
6Shanghai Key Laboratory of Brain Function Restoration and Neural Regeneration, No.12 Mid Wulumuqi Road, Shanghai, 200040, P. R. China
7School of Data and Computer Science, Sun Yat-sen University, No.135 Xingang West Road, Guangzhou, 510275, P. R. China
* Shuo Lu
Email
Email: Lush3@mail.sysu.edu.cn
* Rui Feng
Email: Fengrui@huashan.org.cn
Author Contributions:
S.L. and R.F. designed research; J.Y. and N.K. performed research; N.K. and J.Y. collected data. J.Y. and Z.C. analyzed data; Z.C. contributed new analytic tools; S.L. and R.F. supervised data analysis; J.Y. and S.L. wrote the paper; and R.F, L.G. and N.F.H revised the paper.
Competing Interest Statement:
The authors report no competing interests.
Classification:
Biological science; Neuroscience;
Keywords:
brain tumor, functional plasticity, visual processing, task-state EEG, whole-brain networks
This PDF file includes
Main Text
Figures 1 to 4
Table 1
Abstract
Vision is a key source of information input for humans which involves various cognitive functions and a great range of neural networks inside and beyond the visual cortex. There has been increasing observation that the cognitive outcomes after brain lesion cannot be well predicted by the attributes of the lesion itself, but are influenced by the functional network plasticity. However, the underlying mechanisms of impaired or preserved visual cognitions have not been probed from direct function-execution conditions and few works have observed it on whole-brain dynamic networks. We used high-resolution EEG recordings from 25 patients with brain tumors to track the dynamical patterns of functional reorganization in visual processing tasks with multi-level complexity. By comparing to 24 healthy controls, increased cortical responsiveness as functional compensation was identified in the early phase of processing, which was highly localized to the visual cortex and functional networks and less relevant to the tumor position. In the meanwhile, a wide-spreading enhancement in whole-brain functional connectivity was elicited by the visual word recognition task. We conclude that enhanced early rapid-onset cortical compensation in the local functional networks may contribute to largely preserved basic vision functions and higher-cognitive tasks are vulnerable to impairment but with high sensitivity of functional plasticity being elicited for promising rehabilitation.
Main Text
Introduction
The adult brain has a remarkable plasticity potential after brain damage, which helps to cope with brain lesions and preventing permanent functional deficits (Kolb, Teskey et al. 2010). In the case of brain tumors, plasticity often occurs preoperatively which can delay the onset of functional deficits and enables safer resection of the invaded area (Duffau 2008, Pallud, Capelle et al. 2013, Duffau 2014, Kessel, Baumfalk et al. 2017), and in the meanwhile, complicates the prediction of the postoperative recovery (Kristo, Raemaekers et al. 2015). Hence brain plasticity potential is currently receiving increased attention as its mechanism is vital for preserving the long-term life quality for brain tumor patients.
Given the increasing realization that cognitive functions rely on the interaction of networks instead of isolated activation of brain regions (i.e., studies on after-stroke recovery, reviewed by Cassidy & Cramer, 2017), it is hypothesized that the compensation for disrupted neural functions caused by the brain tumors is made possible by the functional reorganization far-spreading from the tumor location. Numerous reports have described functional recovery after brain tumors involving presumed non- compensable structures (Desmurget, Bonnetblanc et al. 2007, Duffau 2014) which highlights the significance of investigating the visual functional plasticity from the perspective of dynamic interplay within and between neural circuits on a whole-brain scale. In this sense, neural functional plasticity has been poorly explored in brain tumor patients (Cargnelutti, Ius et al. 2020). Previous studies looking into preoperative plasticity of tumor-lesioned brains mainly focused on sensorimotor (Niu, Zhang et al. 2014, Barz, Noack et al. 2018) and language systems(Zhang, Xia et al. 2018). A few fMRI addressed task-based functional connectivity in either the sensorimotor or the language system specifically and found successful adaptive connectivity remodeling in brain tumor patients (Briganti, Sestieri et al. 2012, Li, Dong et al. 2019).
Nevertheless, functional plasticity for visual-related cognitions has rarely been studied, possibly because of the commonly retaining of vision or the technical difficulty of exploring the prompt visual processing. As a key source of information input, vision is crucial for human living and is connected to various cognitive functions. Successful visual processing requires not only intact projection from retina to the primary visual cortex, but also involves a globally distributed network, ranging from the occipital cortex to higher-level perception areas in the temporal, parietal and frontal lobe(Goodale and Milner 1992, Tong 2003). Consequently, the visual functional plasticity cannot be simply predicted by the location of brain tumors. Retainment of normal or close-to-normal visual functions was widely found in patients even with brain tumors invading the visual-related areas (Tokumaru, Sakata et al. 2006, Maclean, Fersht et al. 2013). Little research was done specifically addressing the reorganization of visual function networks. A recent work reported 15 patients with good visual function outcomes after pituitary tumor surgery had stronger visual cortex functional connectivity than the poor outcome group(Lang, Ryu et al. 2020) but the reorganization mechanism remains unclear.
Assessing neuroplasticity from the perspective of dynamic functional networks on the whole-brain scale can be challenging for the techniques. The majority of previous studies looked for preoperative changes in functional activities used fMRI that lacked high-temporal resolution or rest-state data which led to vagueness in observing the dynamic characteristics in function processing in real-time (Cargnelutti, Ius et al. 2020).
In this study, we used millisecond-resolution high-density electroencephalogram (HD-EEG) to examine dynamic functional plasticity in the visual processing of stimuli with hierarchical processing complexity for preoperative brain tumor patients. Considering the diversity of visual-related cognitive processing, we employed three types of stimuli including Chinese characters, human faces and objects in the visual task which are hierarchical regarding processing complexity and neural-network patterns(Rossion, Dricot et al. 2000, Itier and Taylor 2004). The neural functional responses were investigated on the whole-brain scale instead of prior designated regions through multiple task-related analyses, including event-related potentials (ERPs), task-activated source localization and functional connectivities (FCs). Ultimately, this novel approach allowed us to reveal that brain tumors caused compensatory reorganization local to the visual cortex and the maintained ventral-stream visual network during the early-stage processing, which accounts for the largely preserved primary vision functions. More interestingly, high-level visual processing, i.e., visual word recognition, induced a much broader enhancement both in the cortices beyond the stream and in the whole-brain FCs, which highlights susceptibility for higher-level cognition impairments and sensitive rehabilitation potentials.
Materials and Methods
Design and Participants
This study involved HD-EEG task-state experiments of patients with brain tumor before surgeries and comparative analysis with healthy controls. Twenty-five patients with brain tumors and 24 healthy controls were enrolled in the study. Patients were recruited from the department of neurosurgery, Huashan hospital, Fudan University. The inclusion criteria were: (1) Patients with intra-axial brain lesion; (2) strongly right-handed according to the Edinburgh Handedness Inventory ; (3) normal or corrected-to-normal vision; (4) growing up in a Mandarin-speaking environment with Chinese as their first language; (5) cooperating in the completion of EEG tasks. The 24 healthy adults were enrolled at Sun Yat-sen University and Huashan hospital and were all strongly right-handed, with normal or corrected-to-normal vision and growing up in a Mandarin environment with Chinese as their first language.
Four patients and three controls were excluded due to excessive artifacts in EEG data. After exclusion, 21 brain tumor patients (age: 18–63 years, mean (M) = 41.14, standard deviation (SD) = 15.09 years; 10 females) and 21 healthy controls (age: 19–58 years, M=30.8, SD = 9.95 years; 14 females) were studied.
Ethics Statement
The study was conducted under the approval of the Ethics Committee of the Huashan Hospital, Fudan University (ID: 2019-582). Informed written consents were obtained for all participants prior to enrollment in keeping with the Declaration of Helsinki.
Examination of tumor features
Presurgical clinical assessments were conducted by a neurosurgical expert blinded to experiment results and reviewed by another expert. T1 Mprage, T1 contrasted Mprage or T2 flair anatomical MRI images were obtained before surgery and imported in the Brainlab software (https://www.brainlab.com) to reconstruct 3D brain models to locate the focus side. Next, the brain area volume of interest (VOI) for each patient was calculated by Brainlab built-in toolkit based on the basis of signal abnormalities in the 3D brain model by two neurosurgical experts. The pathological diagnosis and grade of the lesions were confirmed by two other neuropathologists. According to the diagnosis and grade examination, tumors of grade I and II were indentified as low-grade tumors(LGT) and those of grade III and IV as high-grade tumors(HGT).
Among the 21 patients, 19 had unilateral lesions (left=6, right=13), and two bilateral lesions. In all patients, the brain tumor volume ranged from 0.61 to 77.9 cm3 (M=25.05, SD=23.08). Detailed information on brain tumor location and volume is aggregated in Supplementary Table 1.
EEG Experiment
Stimuli
Stimuli were 90 gray-scale pictures of Chinese characters, human faces, and objects (Supplementary Fig. 1A). Thirty Asian faces, balanced in male vs. female and children vs. adults, were created as the stimuli for human face condition according to the criteria of the Facial Action Coding System (FACS) (Ekman and Friesen 2002), and all faces were emotionally neutral without any obvious facial expressions. Stimuli materials for the Chinese-character task were selected from “Commonly used Characters table” issued by State Language Affairs Commission, China, and the orthography was controlled on the structure and stroke number to expel possible influence to the neural response. Stimuli for the object task included photos of commonly found animals, food, daily necessaries, etc.
Task procedure
The experimental procedure was programmed with E-Prime 2.0 software. The experimental instructions were first presented on the screen, and the EEG data of a 3-minute rest was collected to ensure that the participants were in a stable condition. The participants were instructed to stare at the fixation point on the screen to maintain their attention. Next, picture stimuli were randomly presented on the screen for 500ms with random intervals of 400-600ms. An example of task procedure and stimuli was shown in Supplementary Fig. 1B and 1C.
Data recording
Participants were seated 80-100 cm away from the stimulus presentation screen in a dimly lit, sound-attenuated room. During the experiment, An EEG Geodesic Sensor Net (GSN)(Tucker 1993, Tucker, Liotti et al. 1994) with 256 evenly distributed sensors were used to record EEG activity from the participants’ scalp (Geodesic EEG System 400, Electrical Geodesics Inc., Eugene, OR, USA), referenced online to the vertex (Cz). All electrode impedances were kept below 40 kΩ throughout the experiment to ensure an optimal signal-to-noise ratio(PICTON, T. et al. 2000, Ferree, Eriksen et al. 2001). The amplified signals were recorded with Net Station software at a sampling rate of 1000 Hz and stored for further offline analyses.
EEG data processing
Preprocessing
The continuous EEG data obtained in this study was offline filtered (1-30 Hz) and re-referenced to an average using the EEGLAB toolbox (Version 2020.0) (Delorme and Makeig 2004), an electrophysiological signal processing toolbox applied to MATLAB (Version 2018b). To improve the signal-to-noise ratio, the “Clean Rawdata and ASR” tool in EEGLAB was used to exclude severely artifacted channels due to head moving, eye blink, eye movement and other physiological reasons. Further, independent component analysis (ICA) with RUNICA algorithm was conducted to remove artifact ICs. Then, the cleaned continuous EEG data were epoched into 700 ms segments (from -100 ms to 600 ms relative to the stimulus onset) and baseline corrected (-100 ms to 0 ms).
Event-Related Potential (ERP) analysis
After preprocessing, EEG epochs were averaged separately for each condition. The key data regarding latency and amplitude of the ERP was measured. For the ERP waveforms, the mean amplitude of each condition was calculated over each successive 20-ms time window from 0 to 600 ms after the stimulus onset, to identify the target ERP components: P1, N170 and P2. The time window where the peak amplitude was located was identified as the peak latency of each component. Electrodes were grouped into eight regions of interest (ROIs) as follows: Left frontal (F3), right frontal (F4), left central (C3), right central (C4), left occipital (O1), right occipital (O2), left posterior temporal (P7), and right posterior temporal (P8). The electrode points and corresponding brain regions distributions are shown in Supplementary Fig. 2. The ERP waveforms of the electrodes in each ROI were averaged, producing a single amplitude and latency value in each time window.
Task-activated Electrical Source Imaging
The task-activated EEG epochs were analyzed for detecting the sources of the neural responses. Source analysis was conducted through GeoSource (EGI Inc.) with the standardized Low-Resolution Electrical Tomography (sLORETA) algorithms, which has zero localization error in noise-free simulations and was widely used in studies of cognitive and perceptual processing(Pascual-Marqui 2002). The source imaging of task-state brain activities used a finite difference method (FDM) realistic head model with 1732 dipoles and the Montreal Neurological Institutes (MNI) typical head image as a template. The source localization was based on functional brain region parcellations, and the automated anatomical labeling (AAL) parcellation with 90 brain regions across the whole brain was adopted (Tzourio-Mazoyer, Landeau et al. 2002). Also, the dipoles in each brain region were summed to represent the source power of each AAL region. An average filter (with a 20ms time window size) is adopted to alleviate some inherent source noises (which exist in most source algorithms). The top 40% of the activated regions, with the dipoles with particularly low power(<0.5*highest power) left out, were identified as highly activated cortical regions. The source imaging and later functional connectivity results were visualized with the BrainNet Viewer (http://www.nitrc.org/projects/bnv/)(Xia, Wang et al. 2013).
Statistical analyses
Statistical analyses were carried out with SPSS 19 (IBM, New York, USA). Independent-samples t-test was performed to compare the latency and amplitude of each ERP with respect to brain regions and conditions. The neural response data were analyzed by one-way ANOVA, with Greenhouse Geisser epsilon being applied to reduce the degree of freedom and to control the variance-nonuniformity related type I errors. The comparison analyses for task-activated sources were performed on basis of region-wise and timestep-wise t-tests, and the critical value for a significance level of 5% was established by a cluster-based permutation tests method using 10000 randomizations (Cheol, E. et al. 2013, Sassenhagen, Blything et al. 2018) Pearson’s correlation and linear regression analyses were used to analyze the correlations between tumor volume and neural activities. P < 0.05 was considered statistically significant, where the p-value was acquired by cluster-based permutation tests method (using 10000 randomizations) with a significance level of 5%, similar to previous work(Cheol, E. et al. 2013, Sassenhagen, Blything et al. 2018). We performed two-way ANOVAs with the factor Electrode (F3, F4, C3, C4, O1, O2, P7 and P8) and conditions (Character, human face and object) for amplitude and latency values separately. Supplementary Fig. 3 presents an overview of the processing pipeline.
Data availability
The data that support the findings of this study is available on request from the corresponding author. The data is not publicly available because of containing information that could compromise the privacy of research participants and can only be accessed under the consent of the participants. In-house scripts have been uploaded as Supplementary material.
Results
To reveal dynamic neural responses to the visual recognition tasks, EEG data from both groups were epoched and averaged across experiment trials to extract response features. Then, to locate the task-activated functional areas and the compensatory topography, dynamic activities on source level were tracked and it was found that brain tumor patients shared a very similar functional network (the ventral stream of visual processing) as controls but had a series of stronger activation in regions local or adjacent to the stream. Furthermore, we also probed into how the whole-brain connectivity changed in the patients, and the results revealed a stronger FC level than controls in multiple extents. The above-mentioned results were all further analyzed to explore their correlations with individual tumor features, and significant sensitivity to tumor volume and grade were discovered in a series of activation responses.
Tumor features: grade,
location and volume
Among the 21 patients, 19 had unilateral lesions (left=6, right=13), and two bilateral lesions. In all patients, the brain tumor volume ranged from 0.61 to 77.9 cm3 (M=25.05, SD=23.08). Detailed information on tumor location and volume is aggregated in Supplementary Table 1.
ERPs
To characterize the alternations of neural responses to visual stimuli in patients along time course, the amplitudes and latencies of the major components in visual processing (P1, N1, N170, VPP and P2) were manually identified by each ROI for both groups and compared between groups. The grand average ERPs wave, P1, N170 and P2 on temporal occipital areas, and N1 and VPP on frontal-parietal areas are shown as representatives in Fig. 1.
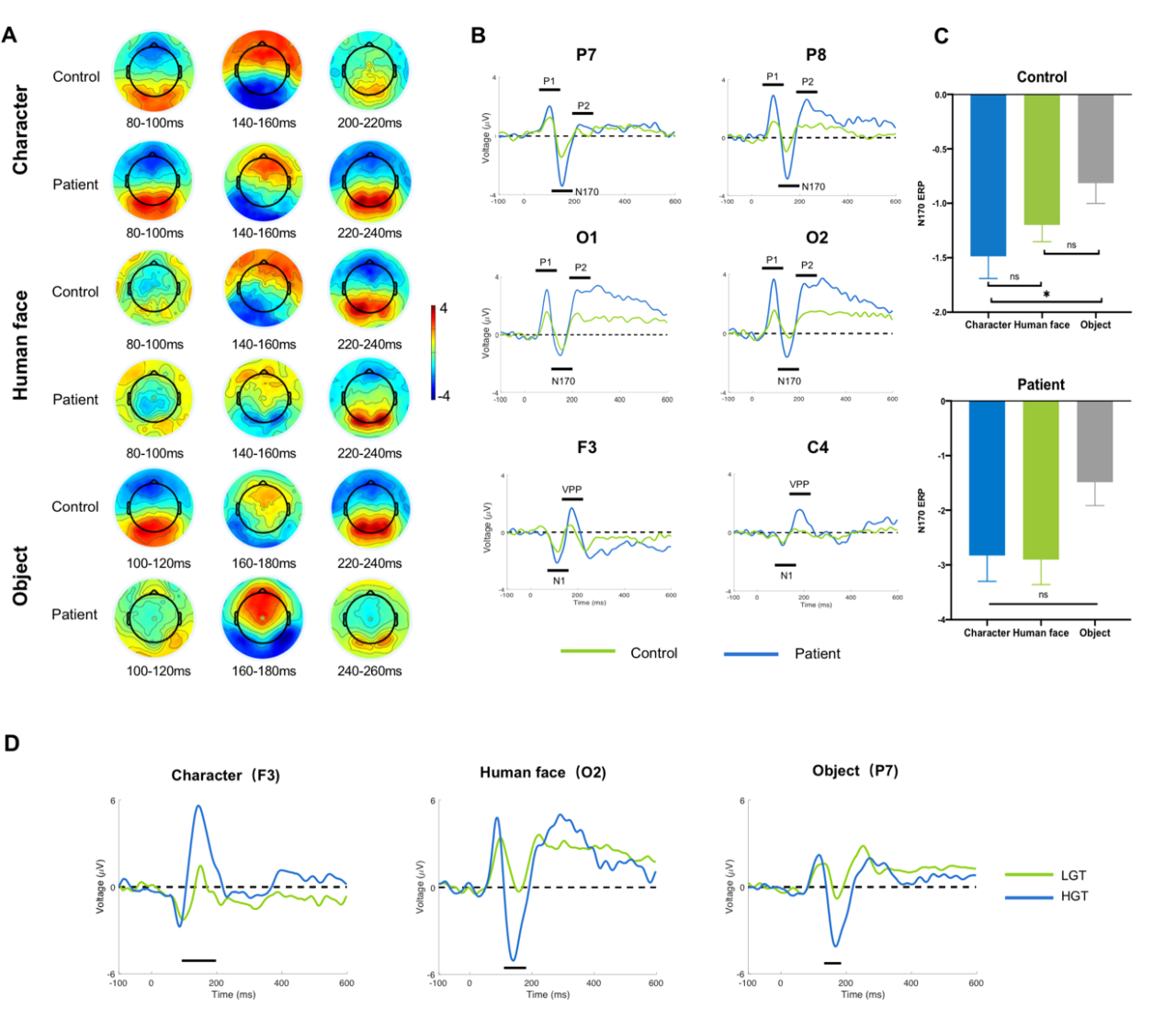
Figure 2. Temporal ERP Response for all three conditions. (A)
Topographic distribution of ERPs of P1/N1, N170/VPP and P2 components triggered by character, human face and object stimuli in the peak time window. (B) Contrasts of Grand-average ERP waveforms between controls and patients over selected ROIs of electrodes (for detailed ROI information, see Supplementary Figure 2). Black lines below the waveforms indicate the recognized ERPs. (C) Main effect of condition in N170 amplitudes. Stars in the figure indicate statistically significant differences and error bars display standard errors of the mean (p < 0.05, running t-test, FDR corrected). (D) Grand-average ERP waveforms on three conditions and representative ROIs for comparison between LGT and LGT patients. Black lines below the waveforms indicate that the amplitudes of control and patients’ waveform are statistically different (t-test, P < 0.05, FDR corrected).
Between-group comparisonof
the ERP amplitudes and latencies
In this analysis, multiple time windows were found to show significant differences in the amplitudes but not latencies between the patient and control group (an exhaustive list is presented in Supplementary Table 2). Under each ROI, condition, and components, amplitudes of patients were generally significantly larger than controls with no significant group effect on most latencies of P1, N1, N170, VPP and P2, except for only VPP of face condition, C3 area (t-test, t=3.299, PFDR < 0.01) and N170 of object condition on O1 area (t-test, t=2.914, PFDR < 0.01). The results revealed generally increased task responses in patients than controls, but the response speed was not significantly affected.
In the control group, a significant main effect of condition was found in the N170 amplitudes on occipital-temporal regions (Two-way ANOVA, F=3.353, PFDR < 0.05) with no interaction effect between conditions and ROIs. In posthoc multiple comparison, amplitudes of character condition were significantly larger than those of object condition (P < 0.05), which revealed the ‘N170 specialization effect’ that was generally found on word print processing when comparing to other types of visual-stimuli perception. However, no difference in N170 amplitudes was found for the patients among the three conditions, indicating an absence of N170 specialization for visual word processing (see Fig. 1C).
Within patient group:
influences of tumor volume and grade
Tumor volumes were found to have a significant correlation with peak amplitudes of the ERPs. For early components P1, N170 and VPP, the correlation was positive, suggesting the larger the tumor was, the higher the wave peak achieved. But for P2, the correlation was negative, indicating that the larger the tumor was, the lower the P2 peak amplitude reached. The correlation curves of P1, N170, and P2 are presented in Supplementary Fig. 4. For the impact of tumor grade, multiple time windows were found to show a significant difference in the amplitudes between HGT and LGT patients in early components (N1, P1, VPP and N170). Under each ROI, condition, components and amplitudes of HGT patients were significantly larger than LGT (see Supplementary Table 2 and waveforms on all conditions on representative ROIs in Fig. 1D). The results suggest the neural arousal in patients is sensitive to tumor volume and grade.
Taken together, the ERPs results reveal for the first time that the plasticity induced by brain tumors in visual functions manifested as stronger but not slower responses in patients than controls, which normally indicates a stronger neuron population and synchronizing activity.
Task-activated source activity
Localization of functional response regions
Since an enhanced neural response was found in the ERP data, the task-activated source activity became necessary to be investigated to reveal the EEG sources of functional response regions to further identified the topography of neural activity alternations in patients. Sensor density of up to 256 electrodes in this study enabled a possibility of high spatial resolution (Thrane, Ulrich et al. 2017) along the time course of functional responses.
The highly activated cortical regions (definition see section “Task-activated Electrical Source Imaging” in method) participating in the visual processing in the two groups were highly overlapped across all three conditions and revealed a shared visual network. The most highly activated regions included bilateral inferior temporal gyri (ITG), bilateral fusiform gyri and left lingual gyrus, which all belong to the ventral stream of visual processing and are critical areas accounting for visual stimuli recognition. Besides the shared ventral stream areas, patients had more task-activated core regions that were not found in control (region-wise t-test, P < 0.05), those regions are AAL 7 (Left frontal middle gyrus) and AAL40 (ParaHippocampal_R) (see Fig. 2A), which might serve as extra compensational sources.
Whole-brain dynamic
change of patient group
A time-wise slide-window test was performed on the source power of every region to find tthe dynamic difference between two groups on task-activated source activities. The results showed that for a group of source regions, patients had significantly stronger electrical power than controls (see Supplementary Table 3), indicating that all three tasks brought greater activation in multiple regions in patients, which is in line with the results of larger amplitudes of ERPs by patients than controls. Specifically, on all the functional response regions except left Lingual, the patients had significant higher source power than controls (time-wise t-test, P < 0.05), which indicated a general enhancement within the visual functional network. For the regions that showed significantly higher source power in patients’ dynamic information was revealed as the patients’ functional reorganization patterns, i.e., areas in the visual cortex were activated in relatively early stages (before 350ms), most regions in the ventral stream were activated in early and middle stages (0ms-500ms), and most frontal areas were activated in late time windows (around 450ms or later). See Fig. 2A and details in Supplementary Table 3.
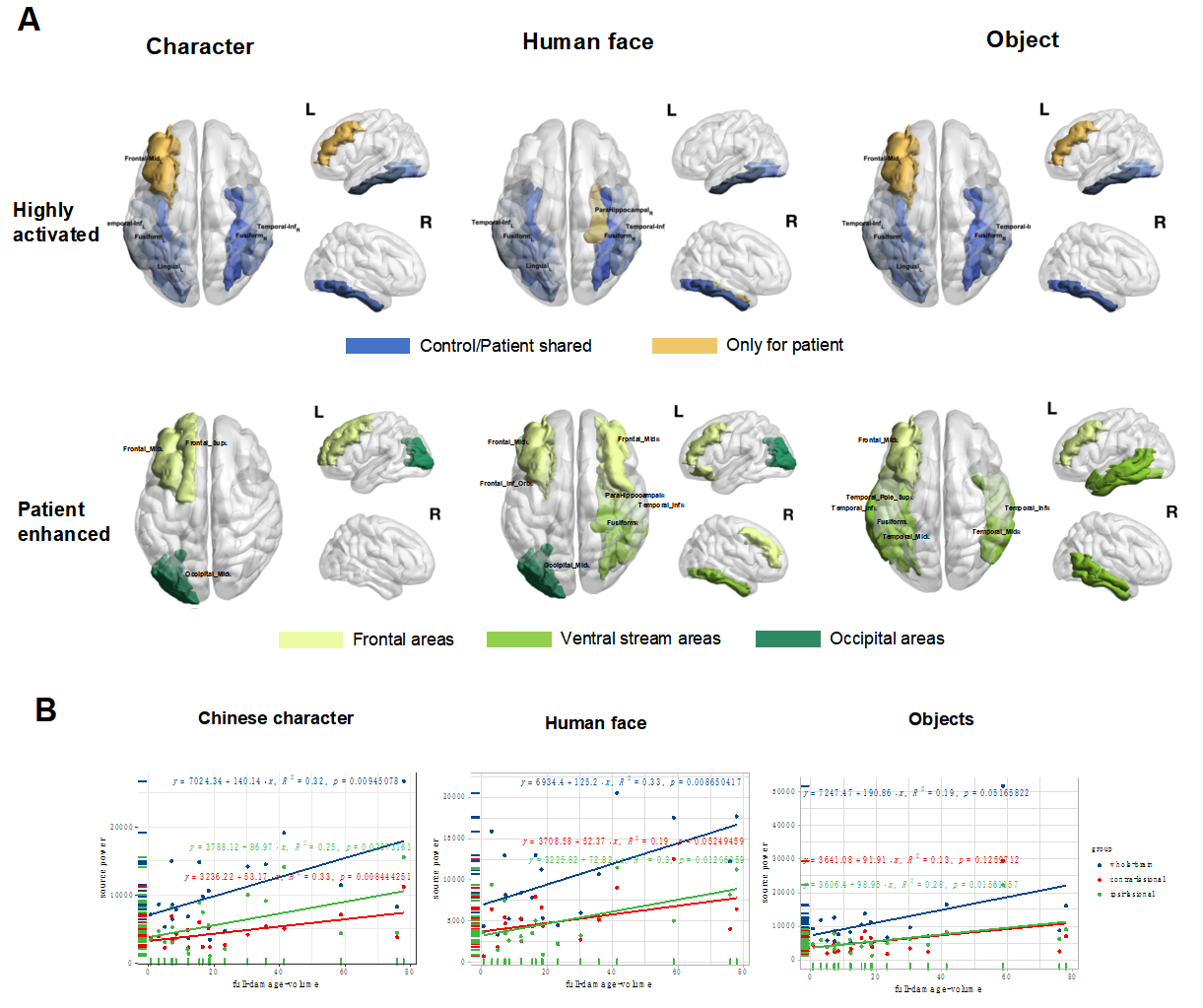
Figure 2. Core regions of task-activated source localization regions for controls and patients under three conditions. (A)
Highly activated core regions (core regions definition: a. leave out the brain regions of lowest 50% of source power for meaninglessly weak activation; b. in remaining areas, find brain areas of top 40% source power) were visualized in the brain map. The blue color indicates regions that are highly activated in both controls and patients, and regions in yellow are highly activated only in patients. The three columns display the conditions. Brain regions that were tested enhanced in patients are visualized in the second row, with different colors indicating which type of functional area or network the regions belong. Names of regions are labeled in the axial-view figures. (B) Linear regression of tumor volume and whole-brain, ipsi-lesional hemisphere and contralesional hemisphere source power. Across patients, the whole-brain, ipsi-lesional hemisphere and contralesional hemisphere source power from character condition, whole-brain and contra-lesional hemisphere source power from human face condition, and ipsilesional hemisphere power from object condition were found significantly correlated with tumor volume values (Linear regression, P < 0.05). The character condition evoked the most sensitive network alternations on a local and far-spreading scale.
Within Patient group:
influence of tumor volume and grade
To detect the possible influence of tumor location, volume and grade on source EEG, ipsi-lesional, contra-lesional hemispheric as well as whole-brain source powers were calculated by summing source powers from all regions inside.
Similar to the association being found in the ERPs amplitudes, multiple significant positive correlations were found between the volume of tumors and source power on both whole-brain level and hemispheric level, suggesting larger tumors tended to induce greater source power activation by the stimuli. Interaction effect of lesion volume and condition was found on the source powers. Most significant results were found under the character condition, with the tumor volume positively correlated to whole-brain/global source powers, as well as ipsilesional and contralesional source powers. While in face condition, significant correlations were found between the volume of tumors and both whole-brain and ipsilesional hemisphere. Yet in the object condition, only the correlations between the volume of tumors and ipsilesional hemisphere were found significant (see Figure 2B). The result indicated a higher sensitivity to tumor volume in character condition, especially across whole-brain networks.
For the influence of tumor grade, higher source powers in HGT than LGT patients were found in a wide range of brain regions. In all three conditions, HGT showed significantly higher source activation than LGT patients in up to 21 regions while only 3 regions showed stronger source powers by LGT than HGT patients. Detailed comparisons are listed in Supplementary Table 4.
Source-level FCs
Whole-brain
FC enhancement in character condition
The visual cognitive tasks were processed not just in isolated brain regions but via dynamic networks which involved the connectivities among the functional regions. To investigate the influence of brain tumors on task-state FCs, we compared FC intensity (the connectivity indexes between each pair of AAL regions) between patients and controls. No connections tested significantly different between groups in the face and object conditions. By contrast, a large number of significantly different connections were found in character condition (Pairs with P<0.05, cluster-based permutation corrected, see Fig. 3A and 3B).
Besides, condition effects were different in the two groups. In controls, few connections were tested significantly different between conditions, but in patients, the Character condition had a great number of connections significantly different from the human face and object condition, with all the connections showing a higher power in character condition (Fig. 3C shows the FCs with significant between-condition differences). These results revealed that brain tumors caused a particular FC-level enhancement on the whole-brain scale when processing the task of visual word recognition.
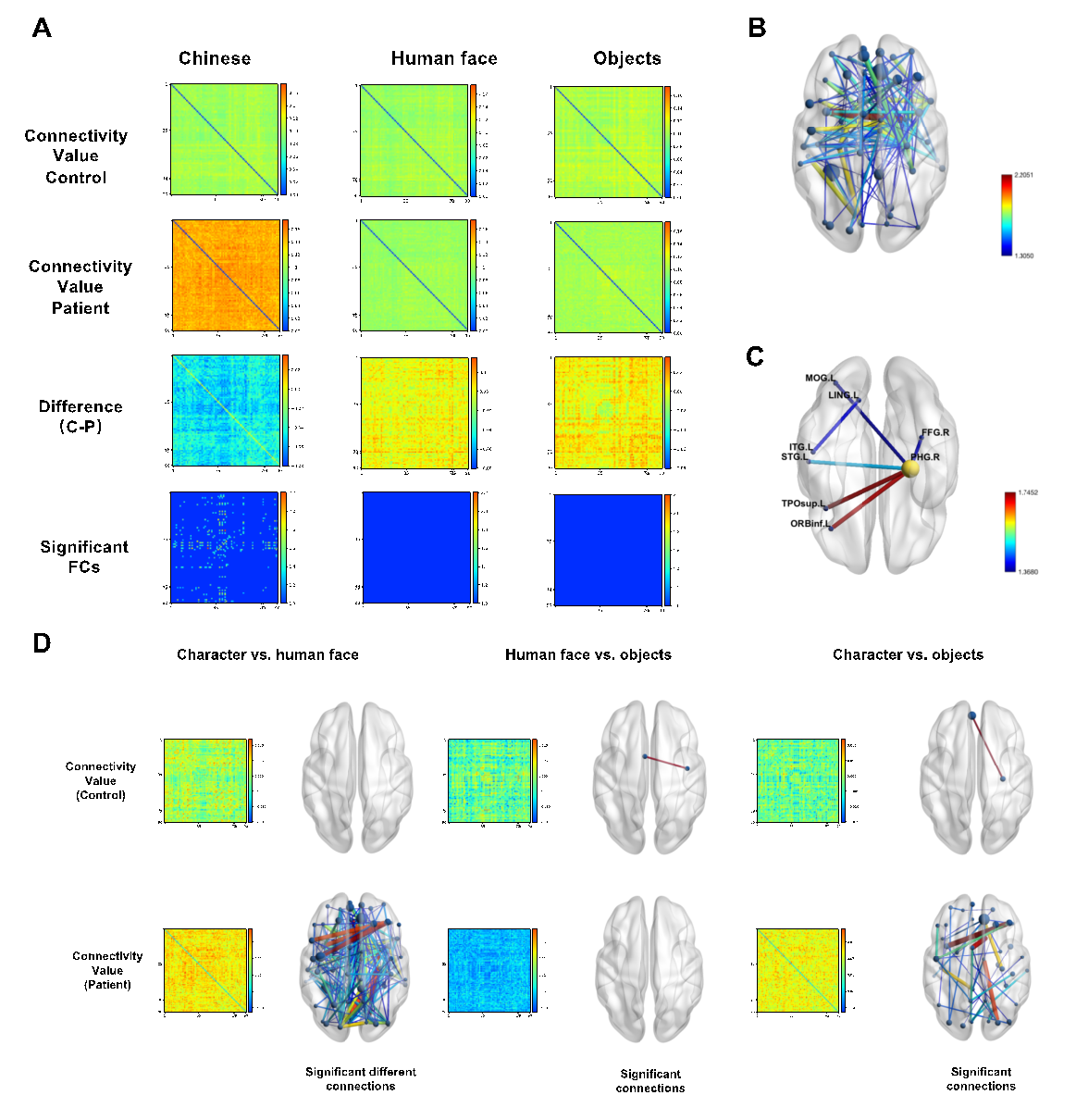
Figure 3. Group comparisons on FC intensity. (A)Group comparison of FCs on three conditions between all brain regions. All dwPLI connectivity values of between-region FCs are depicted on this heatmap, with both the X and Y axis indicating the number of AAL regions and the color bar indicating the strength of FCs. From top to bottom, the rows present connectivity value of controls, connectivity value of patients, the difference value via controls’ FC value minus patients’ FC value, and the connections tested significantly different between groups. Only under the character condition, there are connections found to be significantly different between the two groups of subjects (p < 0.05, cluster-based permutation corrected).(B)The FCs found to be significantly different between group are mapped in the axial view of the brain. The color of each connection indicates .(C)FCs tested to show significant differences between groups within the visual network. The color bar indicates the strength of FCs.(D)FC value and brain mapping of conditions compare. FC levels are shown by color in the heatmap figures of the control and patient group. And statistically significant connections (p < 0.05) are mapped in the right-side axial-view brains. The color of each connection indicates . The character condition task triggered much stronger FCs than the other two conditions only in patients.
FC alternations inside
the visual network
To further address the network alternation in patients, FCs were calculated between each pair of AAL regions from significantly activated source regions shared by two groups as well as group-compared enhanced regions that were identified in the sourcing analysis. Six pairs of FCs were found to be significant in group compare tests. Notice the AAL40 ParaHippocampal_R took a node in five of all the six FCs, suggesting the right parahippocampal area might be a hub node for information communication in visual processing reorganization.
Cognitive performance
Based on neuropsychological self-report and review by clinical experts, the patients were assessed to suffer from multiple cognitive deficits ranging from memory, reading ability, speech communication and emotional health. In all four cognitive performances, there was a higher ratio of deficit in HGT than in LGT patients (see Table 1).
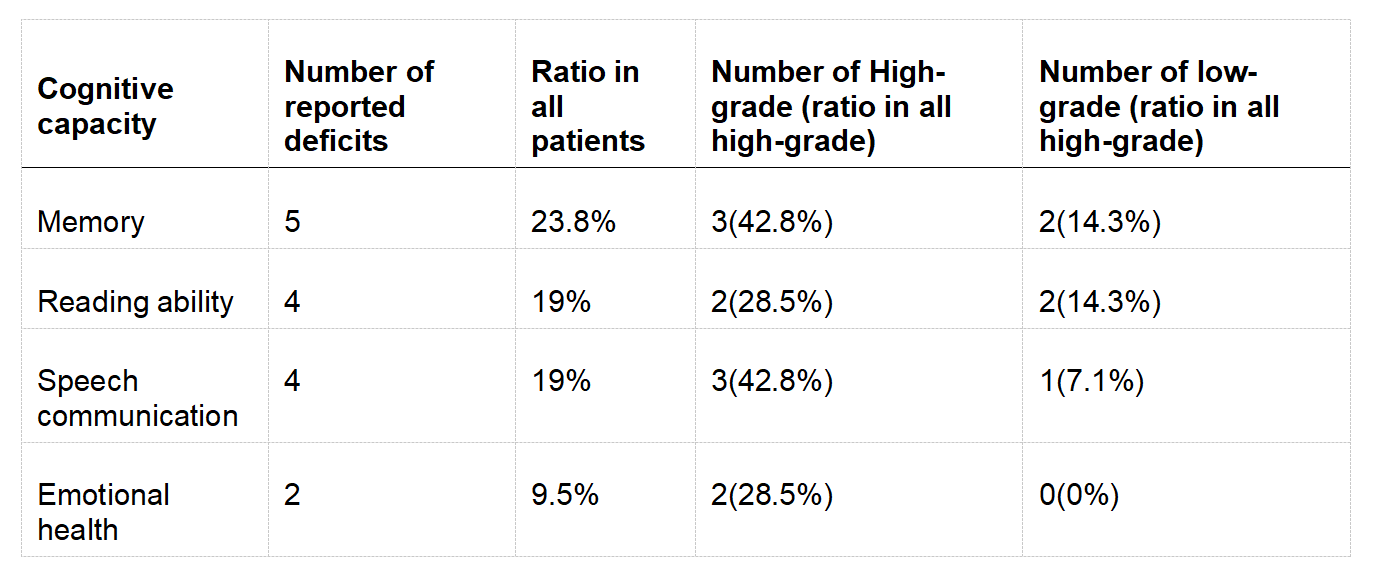
Table 1. Neuropsychological self-report on cognition performances.
Discussion
We examined neural responses to varying types of visual stimuli and characterized functional plasticity in patients with brain tumors via comparison with healthy participants. The tumors induced enhanced neural activities in the visual cortex and visual functional network with no significant impact on processing speed. The compensatory neural activities were identified in early-stage processing with enhanced functional connectivities more localized to the ventral stream and a hub-like node was identified on the right parahippocampal area. Higher-cognitive visual processing elicited particularly enhanced functional connectivities across the whole brain. For the first time, dynamic functional neural activities in task state were investigated across whole-brain networks, which provided insightful observations that characterized the plasticity sensitivity both inside the functional cortex and across the global networks. The findings shed light on operative and postoperative treatments that better prevent permanent visual functional deficits.
Brain tumors induced enhanced
neural activation in a
tumor-volume-dependent manner
in the early stage of processing
The amplitudes of early ERPs (P1, N1, and N170/VPP) and corresponding source powers evoked by the visual processing tasks were found generally greater in patients than in controls under all three conditions, which confirms that brain tumors caused increased neural functional activation. The occipital-temporal P1 and frontal-parietal N1 waves are obligatory, exogenous sensory responses for visual stimuli, involving an attention effect that reflects a general-purpose visual discrimination mechanism(Vogel and Luck 2000, Luck and Kappenman 2012). The occipitotemporal negative and frontocentral positive N170/VPP components were recognized as important visual perception components that strongly elicited by certain classes of visual stimuli, such as faces and words, which were hypothesized to demonstrate perceptual expertise effects(Rossion, Joyce et al. 2003, Joyce and Rossion 2005), greater amplitudes usually suggest a more sensitive or effective processing response, and people with low expertise (i.e. dyslexia or primary school children in low grades) showed a lack of N170 specialization or weaker N170(Maurer, Schulz et al. 2011). Since no work has reported promoted visual processing abilities in brain tumor patients, the stronger ERPs responses in the early processing phase were more likely to be an effort for functional compensation, which was also supported by the behavioral evaluation report that none of the patients in this study had disease-related vision decline.
Moreover, a general positive relationship between the volume of the tumor and the intensity of neural activities (converged on the ERPs amplitudes and source powers) in visual processing was identified in patients. This finding suggests that the functional compensation occurred in a tumor-volume-dependent manner: the larger the tumor is, the stronger the compensational responses are, which in turn confirmed the compensatory role of the enhanced neural responses. However, such dependent effect only existed in the early phase of visual processing, as indicated by P1/N1 and N170/VPP. By contrast, a negative correlation was found in P2 time windows between tumor volume and amplitudes (see Fig. 1D). This may suggest that functional plasticity after brain tumor growth can only compensate the early stage of visual processing, linking to basic aspects of vision, but is confined in late-stage processing involving memory, feeling, semantics, etc. (see reports of related deficits in previous literature (Weyer-Jamora, Brie et al. 2021)) and doubtful to help maintain high-level visual cognitions (see Fig. 1A for the topography of the enhanced early ERPs). This speculation is in line with the behavioral evaluation report that numbers of the patients suffered cognitive impairment related to visual processing, including the reading ability (19%, 4/21), speech communication (19%, 4/21) and memory (23.8%, 5/21). To our best knowledge, this is the first time the dynamic imbalance was identified in direct task-state observation for brain tumor patients’ plasticity patterns.
The robust compensation in early-stage visual processing explains the prevalent retaining of primary vision sense in brain tumor patients and suggests promising recovery potential of primary visual processing.
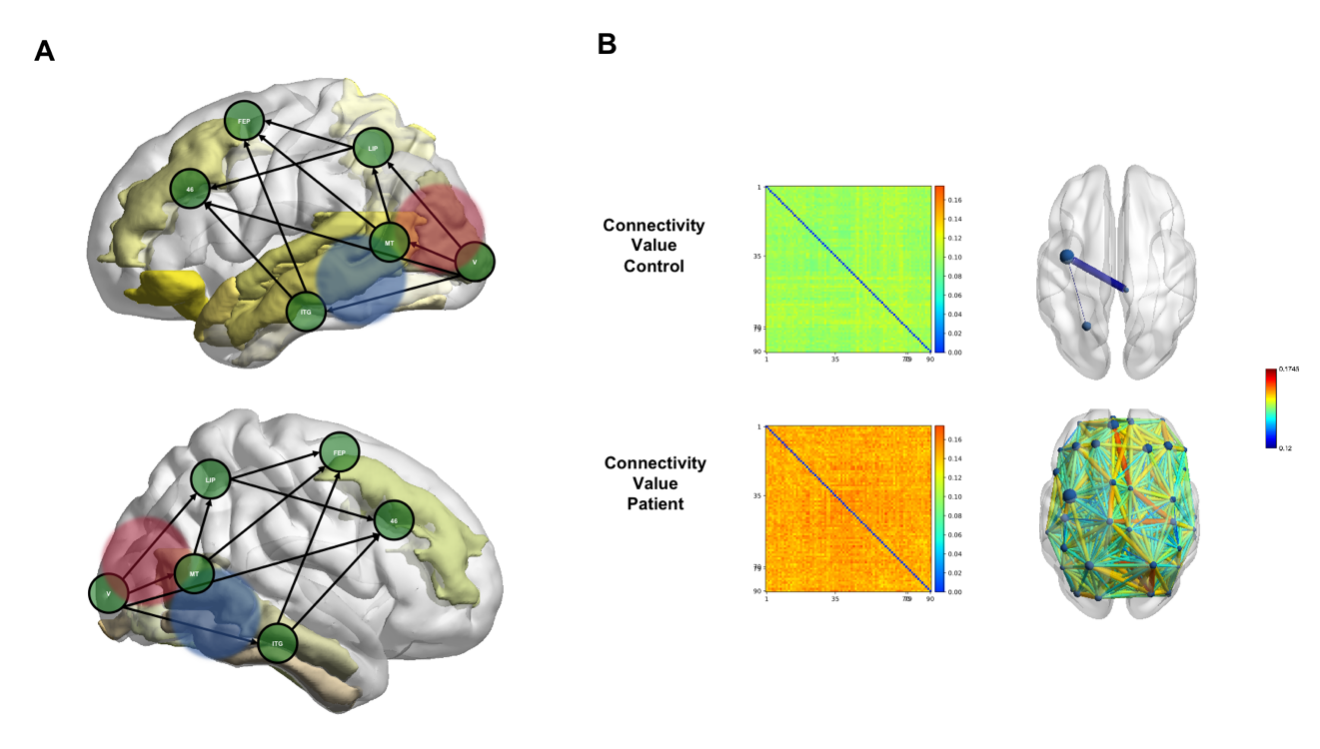
Figure 4. Functional compensation responses in the visual task. (A)Enhanced source activities in local, adjacent and functional-related regions to the two visual streams. The areas in yellow indicate the AAL regions that were found in patients to have enhanced source activities than controls. Visual cortex (V) was found to have reciprocal connections with LIP(lateral intraparietal), the FEP(frontal eye fields) and Brodmann Area 46. The specification of the visual network was cited from (Tong 2003). The red and blue shades indicate the topography of enhanced early ERP components (P1 in red and N170 in blue).(B)FC value and brain mapping of the character condition. FC levels are shown by color in the control group (top row) and in the patient group (bottom row). Connections with dwPLI values larger than 0.12 are mapped in the right-side axial-view brains. The color of each connection indicates dwPLI values of FCs.
However, there are studies that reported decreased neural activities and negative cognition consequences caused by different brain diseases, i.e patients with epilepsy (see section introduction), patients with stroke(Carter, Astafiev et al. 2010, Siegel, Ramsey et al. 2016). Such discrepancy may be explained by the role of disease duration in functional plasticity. As tumors can be seen as relatively slow progressive lesions which may allow the damaged brain function to reorganize in a truly compensatory way, but acute diseases such as stroke or epilepsy with acute discharges will not arise spontaneous neural adaptation in a short time(Kning and Frowein 1988, Mandonnet, Delattre et al. 2003). A similar influence of disease duration on neural plasticity was also observed within post-stroke patients(Liang, Wang et al. 2010). Comparative studies with different types of brain lesions are needed in the future to better understand the mechanism of brain functional plasticity and prognosticate the cognitive consequences.
Functional plasticity is
localized in the visual cortex
and networks
We found the locations of the enhanced neural activities induced by tumors were generally in situ inside the visual functional networks (for visual pathway and networks, see previous works(Tong 2003), although the tumor locations were highly dispersed across the whole brain. The results of ERPs, task-activated sourcing, and FCs converge on this conclusion. First, the significantly larger ERPs amplitudes suggest enhanced synchronization in the local network on the task engagement (Fig. 1). Second, the patients shared very common locations of task-activated sources with the controls located in the canonical visual cortex and functional networks (Fig. 4A). Thirdly, the FC alternations in patients concentrated on the ventral stream of visual networks (Fig. 4B).
It is well documented that brain tumor invasions trigger reorganizations at the whole-brain network level which are not circumscribed to the area invaded by the tumor (Cargnelutti, Ius et al. 2020). By investigating the task-activated neural responses on the whole-brain network, we further confirmed such a pattern and narrowed down the locus of reorganization to the corresponding functional networks. This is the first observation of localized visual functional compensation in the visual networks, although similar functional compensation local in language networks was frequently observed in previous studies (Li, Dong et al. 2019), and on healthy subjects with neural stimulation-induced disruption(Andoh and Paus 2011, Andoh and Zatorre 2013). Similar localized plasticity has also been observed recently on a structural level in brain tumor patients, i.e., structural reshaping of white matter in semantic pathways during a naming task (Amoruso, Geng et al. 2021), grey matter increases in the right hemispheric circuit of language (Zhang, Xia et al. 2018). Zhang and collaborators also reported that structural changes correlated with functional compensation, which highlights the role of functional network responses to deal with the tumor-caused neural plasticity.
It is worth mentioning that a few regions showing significantly higher source power in patients than controls distributed beyond the visual networks. The regions outside the visual networks were all in the frontal lobe (See Fig. 4A) and may represent an effort for attention and executive control which is commonly found in people with brain damage (Puglisi, Sciortino et al. 2019). Another explanation is that these regions participate in higher-level conscious visual perception (Libedinsky and Livingstone 2011), and the enhancement is in line with the localized plasticity in brain tumor patients.
Global-scale plasticity sensitivity
in visual word recognition
It was long before since language-related functions were observed to be compensated in patients with a brain lesion. Previous studies have reported language impairment in patients with brain tumors located inside (Santini, Talacchi et al. 2012) or outside (Lilja, Brun et al. 1992) the language dominant hemisphere. None has been done for detecting the brain tumor patients’ language plasticity specialized to visual word recognition. In the present study, we found the character condition, which called for a visual word recognition task and involved further high-level cognitive processing such as phonology and semantics retrieval, showed particularly high sensitivity in the functional connectivity reorganization comparing to the other two conditions. On the whole-brain scale, we found visual word recognition task-induced enhanced and more-spreading neural activities than other tasks in patients but not controls. Only in character condition, there was a positive correlation between the tumor volume and contra-ipsilesional hemisphere source power. Moreover, only the character processing in which patients had many more connections than controls showed between-group differences on whole-brain FCs (Fig. 4B).
However, results in the present study suggest that the enhanced global FCs in visual word recognition cannot be safely regarded as compensatory. Behaviorally, 4 out of 21 patients reported declined reading ability which is closely dependent on word recognition, although none of them suffered visual impairment. The absence of N170 specialization to character stimuli in patients, which has widely been associated with dyslexia (Mahé, Bonnefond et al. 2012) or illiteracy(Zhao, Li et al. 2012), also implies the patients’ reading deficit. Hence, the above results converge the postulation that the intensity of neural functional reorganizing in brain tumor patients correlates to the complexity of the cognitive function. The higher cognitive functions, though vulnerable to lesion-caused impairment, can evoke more sensitive functional plasticity potential, rendering the brain a possibility for rebuilding the processing networks quickly or compensating related cognitive functions. There is also a possibility that the highly sensitive plasticity is a specific feature of language-related cognitions considering there are extensive observations of language compensation as mentioned above.
In line with a homotopical understanding of brain organization (Catani and ffytche 2005, De Benedictis, Sarubbo et al. 2012, Duffau 2014), functional reallocation would be possible thanks to the existence of redundant cortico-subcortical parallel networks potentially unmasked by the lesion. The wide-spreading FCs sensitivity in word reading helps better understand the mechanism of lesion-caused language plasticity: besides the well-recognized contra-hemisphere or contra-lesional compensation, the globally evoked FCs enhancement is likely to be interpreted as an indicator of functional compensation potential before the compensation actually occurs. The promising recovery prospect is consistent with previous studies that reported functional relevance between increased neural activities in visual processing(Toosy, Werring et al. 2002) and language(Wang, Douw et al. 2010, Andoh and Paus 2011). Although few works have focused on the rehabilitation of text reading in brain tumor patients, the present results suggest the significance of compensatory training focused on reading for an effective reshaping of reading networks and support other related functions.
Nevertheless, there is also the possibility that the high plasticity sensitivity suggests a high risk of related cognitive disorder (i.e., reading difficulty) in the patients. There are studies observing increased networks associated with poorer cognitive functioning in brain tumor patients(Wang, Douw et al. 2010, Derks, Reijneveld et al. 2014), and increased diffuse FCs were linked to reading dysfunction(Zhang, Li et al. 2014). Further longitudinal work is needed to better elucidate the impact of sensitive plasticity in higher-level cognitions on patients’ recovery.
Limitation
First, even though our results show clear evidence of enhanced cortical plasticity in patients, the number of patients investigated in this study was small and not homogeneous enough with regard to age and tumor types, thus requiring caution when generalizing and interpreting current results. Second, the impact of tumor grade was not well investigated due to the limited case number. Generally, the HGT showed a higher level of reorganization with respect to ERPs and task-activated sourcing than the LGT patients. However, in the visual word recognition task, only LGT patients showed significantly stronger FC connections than control (Supplementary Fig. 5 and details in Supplementary Table 4). One hypothesis for this inconsistency is that the reorganization patterns are different regarding the tumor grade and LGT patients have a more sensitive reorganization mechanisms on FCs. This interesting issue will be further addressed in our future studies and left open in the current one. Third, future studies are necessary to replicate the current explorations on postoperative patients and track the functional longitudinal changes to deepen the understanding of brain plasticity mechanism and support more efficient treatment for the patients.
Conclusion
The present studies have identified enhanced neural responses as function compensation in the early stage of visual processing, which suggests promising retaining or recovery of primary visual function. The compensation occurred in situ in the visual cortex and the ventral stream of visual networks irrespectively of tumor location, but the word recognition task-evoked a wide-spreading function connectivity enhancement which is a newly found feature for language plasticity. These findings provide new insights into the plasticity mechanisms caused by tumor invasion, which can help delineate personalized rehabilitation strategies. The characterization of the functional plasticity from a perspective of global cognitive networks with a high-temporal resolution of real-time tracking might open the door to new research avenues. More and more studies have identified the key role of network changes rather than highly localized functional regions to evaluate the cognitive impairment in brain tumor patients(Squire and Zola 1996, Weyer-Jamora, Brie et al. 2021). A clearer characterization of the compensatory brain reorganization in the preoperative period could support safe resection of the invaded areas (Pallud, Capelle et al. 2013). In light of the emerging evidence for improving cognitive functioning using broader cognitive rehabilitation strategies(Stamenova and Levine 2019, Weyer-Jamora, Brie et al. 2021), the therapy of vision-related cognitive functions which are widely distributed with complex network interaction might also benefit from a better understanding of the neural plasticity mechanism globally.
References
Amoruso, L., S. Geng, N. Molinaro, P. Timofeeva, S. Gisbert-Muñoz, S. Gil-Robles, I. Pomposo, I. Quiñones and M. Carreiras (2021). "Oscillatory and structural signatures of language plasticity in brain tumor patients: A longitudinal study." Hum Brain Mapp 42(6): 1777-1793.
Andoh, J. and T. Paus (2011). "Combining functional neuroimaging with off-line brain stimulation: modulation of task-related activity in language areas." J Cogn Neurosci 23(2): 349-361.
Andoh, J. and R. J. Zatorre (2013). "Mapping interhemispheric connectivity using functional MRI after transcranial magnetic stimulation on the human auditory cortex." Neuroimage 79: 162-171.
Barz, A., A. Noack, P. Baumgarten, V. Seifert and M.-T. Forster (2018). "Motor Cortex Reorganization in Patients with Glioma Assessed by Repeated Navigated Transcranial Magnetic Stimulation–A Longitudinal Study." World Neurosurgery 112: e442-e453.
Briganti, C., C. Sestieri, P. A. Mattei, R. Esposito, R. J. Galzio, A. Tartaro, G. L. Romani and M. Caulo (2012). "Reorganization of functional connectivity of the language network in patients with brain gliomas." AJNR Am J Neuroradiol 33(10): 1983-1990.
Cargnelutti, E., T. Ius, M. Skrap and B. Tomasino (2020). "What do we know about pre- and postoperative plasticity in patients with glioma? A review of neuroimaging and intraoperative mapping studies." Neuroimage Clin 28: 102435.
Carter, A. R., S. V. Astafiev, C. E. Lang, L. T. Connor, J. Rengachary, M. J. Strube, D. L. Pope, G. L. Shulman and M. Corbetta (2010). "Resting interhemispheric functional magnetic resonance imaging connectivity predicts performance after stroke." Ann Neurol 67(3): 365-375.
Catani, M. and D. ffytche (2005). "The rises and falls of disconnection syndromes." Brain : a journal of neurology 128: 2224-2239.
Cheol, E., Han, Sang, Wook, Yoo, Sang, Won, Seo and Duk (2013). "Cluster-Based Statistics for Brain Connectivity in Correlation with Behavioral Measures." PLoS ONE 8(8): e72332.
De Benedictis, A., S. Sarubbo and H. Duffau (2012). "Subcortical surgical anatomy of the lateral frontal region: human white matter dissection and correlations with functional insights provided by intraoperative direct brain stimulation: laboratory investigation." J Neurosurg 117(6): 1053-1069.
Delorme, A. and S. Makeig (2004). "EEGLAB: an open source toolbox for analysis of single-trial EEG dynamics including independent component analysis." Journal of Neuroscience Methods 134(1): 9-21.
Derks, J., J. Reijneveld and L. Douw (2014). "Neural network alterations underlie cognitive deficits in brain tumor patients." Current opinion in oncology 26.
Desmurget, M., F. Bonnetblanc and H. Duffau (2007). "Contrasting acute and slow-growing lesions: a new door to brain plasticity." Brain 130(4): 898-914.
Duffau, H. (2008). Brain plasticity and tumors. Advances and Technical Standards in Neurosurgery. J. D. Pickard, N. Akalan, C. Di Rocco et al. Vienna, Springer Vienna: 3-33.
Duffau, H. (2014). "The huge plastic potential of adult brain and the role of connectomics: New insights provided by serial mappings in glioma surgery." Cortex 58: 325-337.
Ekman, P. E. and W. V. Friesen (2002). "Facial action coding system (FACS)."
Ferree, T. C., K. J. Eriksen and D. M. Tucker (2001). "Correction to "Regional head tissue conductivity estimation for improved EEG analysis"." IEEE Transactions on Biomedical Engineering 47(6): 742.
Goodale, M. A. and A. D. Milner (1992). "Separate visual pathways for perception and action." Trends in Neurosciences 15(1): 20-25.
Itier, R. J. and M. J. Taylor (2004). "N170 or N1? Spatiotemporal Differences between Object and Face Processing Using ERPs." Cerebral Cortex 14(2): 132-142.
Joyce, C. and B. Rossion (2005). "The face-sensitive N170 and VPP components manifest the same brain processes: The effect of reference electrode site." Clinical Neurophysiology 116(11): 2613-2631.
Kessel, E. V., A. E. Baumfalk, M. Zandvoort, P. A. Robe and T. J. Snijders (2017). "Tumor-related neurocognitive dysfunction in patients with diffuse glioma: a systematic review of neurocognitive functioning prior to anti-tumor treatment." Springer Open Choice 134(1).
Kning, W. and R. A. Frowein (1988). "Tumor Growth of Recurrent Meningiomas." Springer Berlin Heidelberg.
Kolb, B., G. C. Teskey and R. Gibb (2010). "Factors influencing cerebral plasticity in the normal and injured brain." Front Hum Neurosci 4: 204.
Kristo, G., M. Raemaekers, G. J. Rutten, B. de Gelder and N. F. Ramsey (2015). "Inter-hemispheric language functional reorganization in low-grade glioma patients after tumour surgery." Cortex 64: 235-248.
Lang, S., W. H. A. Ryu, Y. Starreveld and F. Costello (2020). "Good Visual Outcomes After Pituitary Tumor Surgery are Associated With Increased Visual Cortex Functional Connectivity." Journal of Neuro-Ophthalmology Publish Ahead of Print.
Li, Q., J. W. Dong, G. Del Ferraro, N. Petrovich Brennan, K. K. Peck, V. Tabar, H. A. Makse and A. I. Holodny (2019). "Functional Translocation of Broca's Area in a Low-Grade Left Frontal Glioma: Graph Theory Reveals the Novel, Adaptive Network Connectivity." Frontiers in Neurology 10(702).
Liang, Wang, Chunshui, Yu, Hai, Chen, Wen, Qin, Yong and He (2010). "Dynamic functional reorganization of the motor execution network after stroke." Brain: A Journal of Neurology 133(4): 1224-1238.
Libedinsky, C. and M. Livingstone (2011). "Role of prefrontal cortex in conscious visual perception." J Neurosci 31(1): 64-69.
Lilja, Å., A. Brun, L. Salford, R. Öhman, G. Smith, S. Hagstadius and J. Risberg (1992). "Neuropsychological Indexes of a Partial Frontal Syndrome in Patients With Nonfrontal Gliomas." Neuropsychology 6: 315-326.
Luck, S. and E. Kappenman (2012). "ERP Components and Selective Attention." The Oxford handbook of event-related potential components: 295-328.
Maclean, J., N. Fersht, F. Bremner, C. Stacey, S. Sivabalasingham and S. Short (2013). "Meningioma Causing Visual Impairment: Outcomes and Toxicity After Intensity Modulated Radiation Therapy." International Journal of Radiation Oncology*Biology*Physics 85(4): e179-e186.
Mahé, G., A. Bonnefond, N. Gavens, A. Dufour and N. Doignon-Camus (2012). "Impaired visual expertise for print in French adults with dyslexia as shown by N170 tuning." Neuropsychologia 50(14): 3200-3206.
Mandonnet, E., J. Delattre, M. Tanguy, K. R. Swanson, A. F. Carpentier, H. Duffau, P. Cornu, R. V. Effenterre, E. C. Alvord and L. Capelle (2003). "Continuous growth of mean tumor diameter in a subset of grade II gliomas." Annals of Neurology 53(4).
Maurer, U., E. Schulz, S. Brem, S. Mark, K. Bucher, E. Martin and D. Brandeis (2011). "The development of print tuning in children with dyslexia: Evidence from longitudinal ERP data supported by fMRI." Neuroimage 57(3): 714-722.
Niu, C., M. Zhang, Z. Min, N. Rana Magar, Q. Zhang, X. Liu, M. Li and P. Lin (2014). "Motor Network Plasticity and Low-Frequency Oscillations Abnormalities in Patients with Brain Gliomas: A Functional MRI Study." PloS one 9: e96850.
Pallud, J., L. Capelle, L. Taillandier, M. Badoual, H. Duffau and E. Mandonnet (2013). "The silent phase of diffuse low-grade gliomas. Is it when we missed the action?" Acta Neurochir (Wien) 155(12): 2237-2242.
Pascual-Marqui, R. D. (2002). "Standardized low-resolution brain electromagnetic tomography (sLORETA): technical details." Methods Find Exp Clin Pharmacol 24 Suppl D(Suppl D): 5-12.
PICTON, W. T., BENTIN, S., BERG, P., DONCHIN, E., HILLYARD and A. S. (2000). "Guidelines for using human event-related potentials to study cognition: Recording standards and publication criteria." Psychophysiol.
Puglisi, G., T. Sciortino, M. Rossi, A. Leonetti, L. Fornia, M. Conti Nibali, A. Casarotti, F. Pessina, M. Riva, G. Cerri and L. Bello (2019). "Preserving executive functions in nondominant frontal lobe glioma surgery: an intraoperative tool." Journal of Neurosurgery JNS 131(2): 474-480.
Rossion, B., L. Dricot, A. Devolder, J. M. Bodart, M. Crommelinck, B. De Gelder and R. Zoontjes (2000). "Hemispheric asymmetries for whole-based and part-based face processing in the human fusiform gyrus." J Cogn Neurosci 12(5): 793-802.
Rossion, B., C. A. Joyce, G. W. Cottrell and M. J. Tarr (2003). "Early lateralization and orientation tuning for face, word, and object processing in the visual cortex." Neuroimage 20(3): 1609-1624.
Santini, B., A. Talacchi, G. Squintani, F. Casagrande, R. Capasso and G. Miceli (2012). "Cognitive outcome after awake surgery for tumors in language areas." Journal of Neuro-Oncology 108(2): 319-326.
Sassenhagen, J., R. Blything, E. V. M. Lieven and B. Ambridge (2018). "Frequency Sensitivity of Neural Responses to English Verb Argument Structure Violations." Collabra Psychology 4(1): 38.
Siegel, J. S., L. E. Ramsey, A. Z. Snyder, N. V. Metcalf, R. V. Chacko, K. Weinberger, A. Baldassarre, C. D. Hacker, G. L. Shulman and M. Corbetta (2016). "Disruptions of network connectivity predict impairment in multiple behavioral domains after stroke." Proc Natl Acad Sci U S A 113(30): E4367-4376.
Squire, L. R. and S. M. Zola (1996). "Structure and function of declarative and nondeclarative memory systems." Proc Natl Acad Sci U S A 93(24): 13515-13522.
Stamenova, V. and B. Levine (2019). "Effectiveness of goal management training® in improving executive functions: A meta-analysis." Neuropsychol Rehabil 29(10): 1569-1599.
Thrane, F. M., L. Ulrich, V. Kishore, H. Larsson, L. H. Pinborg, T. W. Kj?R, F. Martin, S. Claus, O. Brice and T. Carsten (2017). "Safety and EEG data quality of concurrent high-density EEG and high-speed fMRI at 3 Tesla." Plos One 12(5): e0178409.
Tokumaru, A. M., I. Sakata, H. Terada, S. Kosuda, H. Nawashiro and M. Yoshii (2006). "Optic nerve hyperintensity on T2-weighted images among patients with pituitary macroadenoma: correlation with visual impairment." Ajnr American Journal of Neuroradiology 27(2): 250-254.
Tong, F. (2003). "Primary visual cortex and visual awareness." Nature Reviews Neuroscience 4(3): 219-229.
Toosy, A. T., D. J. Werring, E. T. Bullmore, G. T. Plant and A. J. Thompson (2002). "Functional magnetic resonance imaging of the cortical response to photic stimulation in humans following optic neuritis recovery." Neuroscience Letters 330(3): 255-259.
Tucker, D. M. (1993). "Spatial sampling of head electrical fields: the geodesic sensor net." Electroencephalogr Clin Neurophysiol 87(3): 154-163.
Tucker, D. M., M. Liotti, G. F. Potts, G. S. Russell and M. I. Posner (1994). "Spatiotemporal analysis of brain electrical fields." Human Brain Mapping 1(2).
Tzourio-Mazoyer, N., B. Landeau, D. Papathanassiou, F. Crivello, O. Etard, N. Delcroix, B. Mazoyer and M. Joliot (2002). "Automated anatomical labeling of activations in SPM using a macroscopic anatomical parcellation of the MNI MRI single-subject brain." Neuroimage 15(1): 273-289.
Vogel, E. and S. Luck (2000). "The visual N1 component as an index of a discrimination process." Psychophysiology 37: 190-203.
Wang, H., L. Douw, J. M. Hernández, J. C. Reijneveld, C. J. Stam and P. Van Mieghem (2010). "Effect of tumor resection on the characteristics of functional brain networks." Phys Rev E Stat Nonlin Soft Matter Phys 82(2 Pt 1): 021924.
Weyer-Jamora, C., M. S. Brie, T. L. Luks, E. M. Smith, S. E. Braunstein, J. E. Villanueva-Meyer, P. M. Bracci, S. Chang, S. L. Hervey-Jumper and J. W. Taylor (2021). "Cognitive impact of lower-grade gliomas and strategies for rehabilitation." Neurooncol Pract 8(2): 117-128.
Xia, M., J. Wang, H. Yong and C. Peter (2013). "BrainNet Viewer: A Network Visualization Tool for Human Brain Connectomics." Plos One 8(7): e68910.
Zhang, M., J. Li, C. Chen, G. Xue, Z. Lu, L. Mei, H. Xue, F. Xue, Q. He, C. Chen, M. Wei and Q. Dong (2014). "Resting-state functional connectivity and reading abilities in first and second languages." Neuroimage 84: 546-553.
Zhang, N., M. Xia, T. Qiu, X. Wang, C. P. Lin, Q. Guo, J. Lu, Q. Wu, D. Zhuang, Z. Yu, F. Gong, N. U. Farrukh Hameed, Y. He, J. Wu and L. Zhou (2018). "Reorganization of cerebro-cerebellar circuit in patients with left hemispheric gliomas involving language network: A combined structural and resting-state functional MRI study." Hum Brain Mapp 39(12): 4802-4819.
Zhao, J., S. Li, S. E. Lin, X. H. Cao, S. He and X. C. Weng (2012). "Selectivity of N170 in the left hemisphere as an electrophysiological marker for expertise in reading Chinese." Neurosci Bull 28(5): 577-584.